The path to more widespread adoption of EVs is fraught with many daunting challenges. Right now, OEMs are largely living up to that challenge, pumping out new EV designs and models at an eye-watering pace. We’ll have no shortage of choice, it seems, if and when the day comes that battery electric vehicles (BEVs) are the only vehicles on the market. One challenge that still holds people back, however, is range anxiety.
Range anxiety refers to the fear people have that the car they’re driving will run out of power in the middle of nowhere with no charging point anywhere in sight. At least with a gasoline car in this situation you can try walking to a nearby gas station with a fuel can and bringing back some gasoline. You can’t keep electricity in a can, though, can you? Well, not yet at least.
To look at most media outlets, however, you needn’t worry about range anxiety anymore because the next revolutionary battery technology that will change everything always seems to be just around the corner.
In today’s blog, we’re going to explore some of these future battery technologies, get the real scoop on them, what stage of development they’re at, and if they can really end range anxiety for good.
Background: Why Range Anxiety Matters
Range anxiety remains one of the biggest obstacles to people willingly adopting electric cars, the main reasons together being:
- Cost of EVs too high — even with government incentives
- Range anxiety
- Lack of local charging infrastructure
While costs may be people’s top concern, range anxiety would remain even if the cost of EVs suddenly matched that of regular gasoline cars tomorrow. The number of EVs that can match the total single-gas-tank range of the average sedan is still very low. The average sedan does about 25.7 miles per gallon (according to energy.gov) with a gas tank of about 15 gallons. That’s a total of about 385 miles.
When you factor in hybrids, smaller and more efficient European and Japanese cars, and so on, you end up with a picture in which you can only beat a gasoline car if you have a Tesla or a Lucid Air, neither of which is exactly affordable. The next best option might be a Nissan Leaf or a Chevrolet Bolt, neither of which exceed 300 miles in their total range, even in the 2022 model year.
Range anxiety matters because it’s a real problem that the bulk of people have. Even in a Tesla, there’s just not the same kind of charging/fueling infrastructure safety that there is for gasoline cars. Very few people fear running out of gas because there is seemingly always a gas station within easy range somewhere.
The first part of the cure for range anxiety is better battery technology. The second part is installation of more charging infrastructure. The second part is already underway, so in the next section we’ll take a closer look at the kinds of new and emerging battery technologies that will hopefully cure range anxiety everywhere.
Top 6 New and Noteworthy Battery Technologies
Lithium-Sulfur

Back in 2020, the European Commission published an article relating to research that had been done into lithium-sulfur battery technology. These are batteries that work in a similar method to our current and popular lithium-ion batteries, but instead of costly cobalt, they use sulfur.
This in itself, regardless of other properties, is a more attractive proposition than our current lithium-ion designs that rely on cobalt. The majority of the world’s current supply of cobalt comes from the Democratic Republic of the Congo, and demand is already starting to outstrip the supply from there.
Sulfur, on the other hand, is an abundant material found in many places around the world, and is actually a by-product of the oil industry — perhaps giving a new lease of life to that industry when it’s no longer required to fuel everyone’s car!
The main benefits of lithium-sulfur, besides the abundance of the raw material, is that they have far more potential for energy storage than lithium-ion batteries, so technically they sound ideal to solve the problem of range anxiety.
Numbers are not precise, but imagine that they could double the current ranges of most electric vehicles. That would instantly make EVs as rangeworthy as many regular vehicles, even hybrids. Lithium-sulfur batteries are also lighter and cheaper, which would bring EV prices down.
The main limitation of lithium-sulfur as things stand is that the batteries cannot ensure a high enough number of charging cycles before they give out. This makes them far less viable. A typical Tesla battery can endure about 1,500 charge cycles, but the most current lithium-sulfur batteries can only last 50.
Therefore, this is a great technology for eliminating range anxiety, but unfortunately it would only work in the short term and you’d have to replace the battery too often for it to be a commercially viable product.
Sodium-Ion
Another technology thought to be promising is that of sodium-ion batteries, which operate on the idea of sodium ions being extracted from a cathode and put into an anode during discharge, and vice versa during the charging process. While discharging, electrons would travel through the external electrical circuit, “offering their services” as they went.
The first benefit of the sodium-ion battery, like the sodium-sulfur battery, is that it doesn’t depend on any rare materials that are concentrated in too few places. Lithium-ion is scarce, lead-acid is too toxic, but sodium-ion solves these issues.
They are also far more affordable overall than lithium-ion, which typically cost about $140 per kWh of capacity, compared to just $50 or so for a sodium-ion battery.
On top of those benefits, sodium-ion batteries use safe, non-flammable materials that also work well in colder conditions — good news for the EV drivers of Norway! — but they do suffer from one key flaw, as revealed by extensive research at Cornell University.
Issues with their durability were well known, but it was Cornell scientists who discovered that it was down to what they call an “atomic reshuffling,” where “ions traveling through the battery disorder crystal structures and eventually break them.”
Solid State
As the name suggests, solid-state batteries differ from common lithium-ion batteries in their use of electrolytes. The solid-state batteries use solid layers of electrolytes to carry lithium ions between the electrodes, while lithium-ion batteries make use of liquid electrolytes:
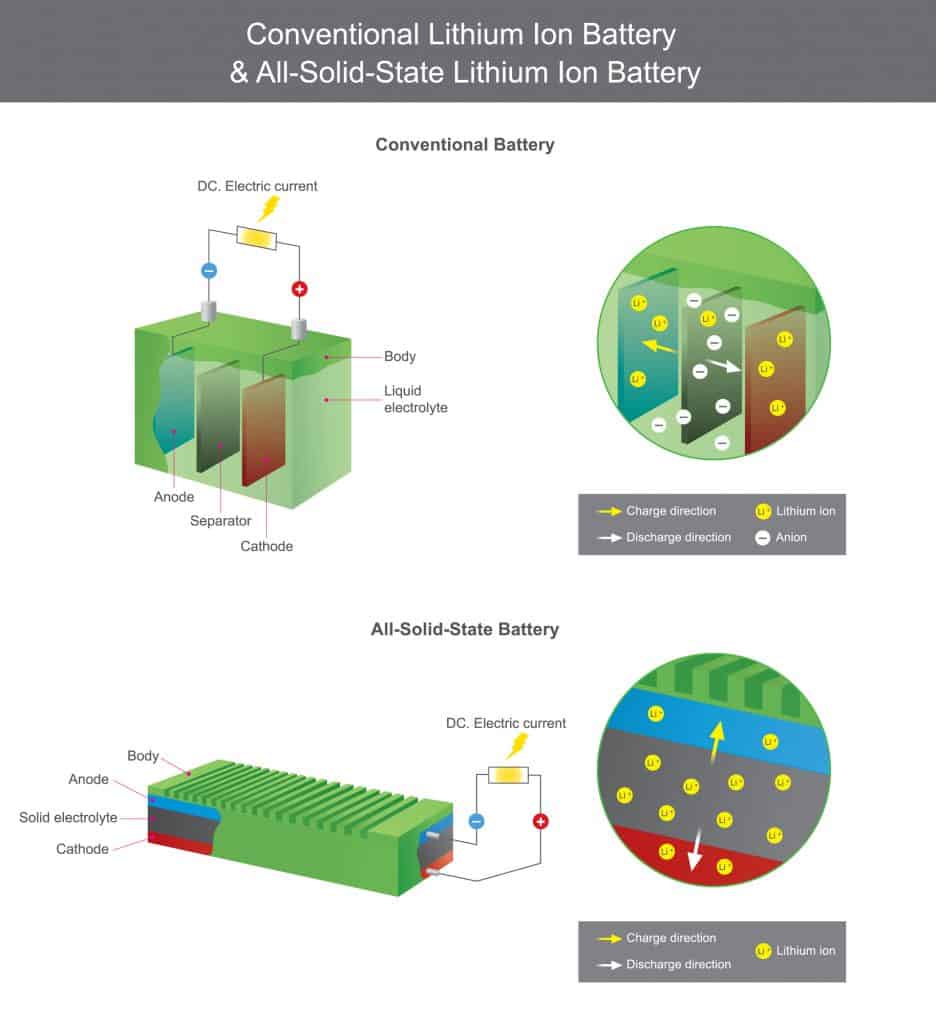
They’re a relatively new technology but already in commercial use, and you’ll find them in many smart watches, as well as medical devices such as pacemakers.
The inherent advantage of solid-state batteries is their stability. The use of liquid electrolytes requires separators and other steps taken to prevent components coming into contact when they shouldn’t. When the batteries reach high temperatures, they lose stability and can even burst into flames, a lesson that Samsung learned very painfully when they released the Galaxy Note 7.
So, what’s the problem with solid-state batteries? If they’re more stable than lithium-ion, why aren’t they rolling off the production lines? In small-scale cases, they are (e.g., above-mentioned smart watches and pacemakers), but in sizes usable in an electric car they have only yet been produced in a lab one at a time.
With hundreds of thousands of EVs already rolling off the production lines, and millions more to come, how viable are solid-state batteries of that scale?
SINANODE
To avoid getting overly technical, let’s take the definition of SINANODE from its main creator and operator, OneD. According to their website, SINANODE is:
“A collection of technologies to produce silicon nanowires fused directly onto the commercial graphite particles used in the anodes of EV batteries. These technologies “supercharge” the amount of energy stored, the speed of charging, and the power delivered”
SINANODE Website, June 2022
OneD claims that SINANODE technology can actually store up to 10 times more energy than graphite, but the main challenge is, similar to solid-state batteries, that the silicon materials are currently produced in too small quantities, and can only be applied at a very limited scale, that currently only modest gains can be made.
Graphene Batteries
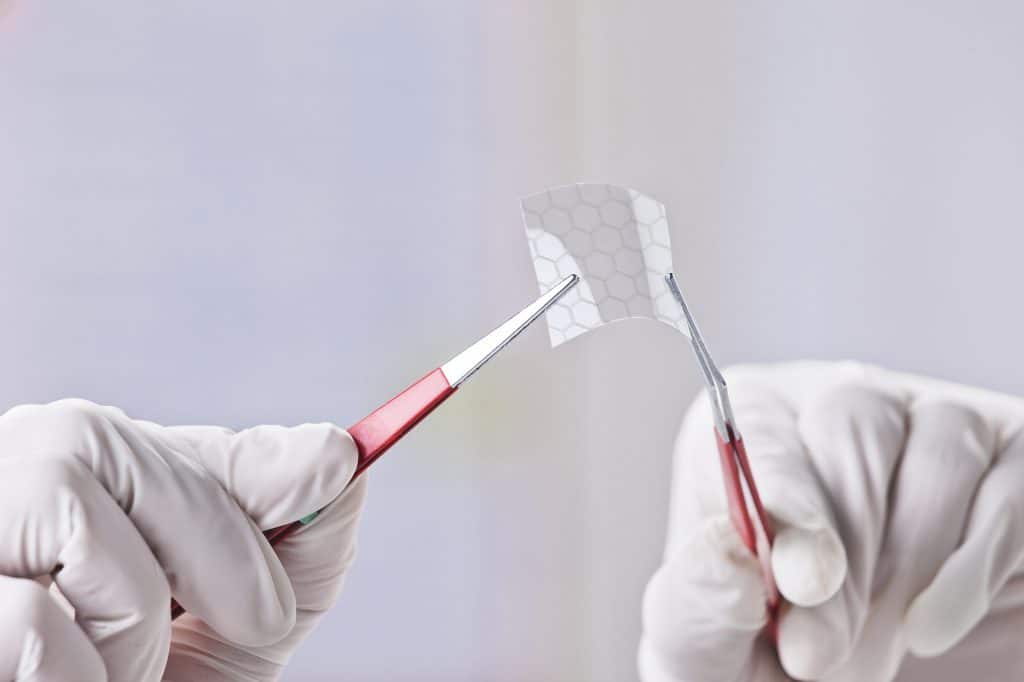
Graphene is seen currently as a material that could be used to drastically improve the performance of existing battery technology, in particular lithium-ion batteries. It can be combined with a number of other materials to create new battery chemistries that benefit the battery performance — as well as its eco-friendly credentials — in a number of different ways.
The key process is to get sufficient graphene into the electrodes to help improve both charging rate and battery capacity.
The main problem, however, is that the more graphene that is used in the anodes, the less stable the output, which is obviously a big roadblock to using the material practically. Graphene is also a very new material, and once again there is no current method known for mass production of the material.
Cobalt-Free Lithium-Ion
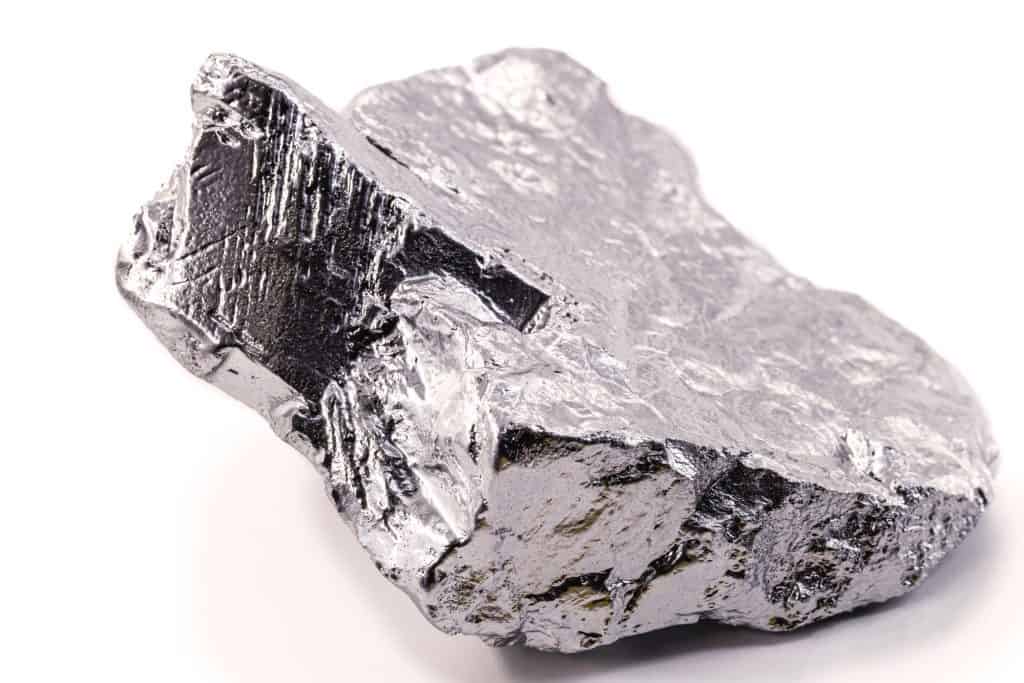
Cobalt is currently one of the most critical elements to making lithium-ion batteries work. However, as we’ve already stated above, it’s incredibly hard and expensive to acquire, and supplies are very uncertain. Cobalt is necessary for creating a high rate of lithium ion delivery to the cathode, but while also maintaining a stable reaction environment that’s less likely to start a fire. But now there might be an alternative, namely Manganese.
Manganese is cheaper at around $1700 a ton — cobalt can be $40,000+ a ton — and the better price tag also means that companies are willing and able to mine it more ethically in places like Canada, for instance.
A $40,000 a ton price tag means you will more likely turn to the unstable and desperate regions of the world, even if it’s riskier and means the locals are worse off. That’s just how so much of international business works. Manganese is also cheaper than some other alternatives such as nickel.
So, what’s the big drawback? The main issue, as with graphene, is stability. When you increase the levels of manganese and lithium, the inevitable trade-off is a decreased level of stability in the cathode. With the right balance, however, manganese is arguably the most promising material solution that we have, currently.
Conclusion: Is Range Anxiety Over? Not Yet
It seems that range anxiety is still with us to stay for the moment. There are clearly some great options on the horizon, but it will take us some time to get to a place where these are commercially viable.
Most experts agree that we won’t be able to rely on a single technology to meet our energy storage needs when it comes to EVs and the plethora of other electronic devices that rely on similar rechargeable battery technology. Therefore, perhaps we’ll be waiting for all of the above to come to fruition, and then some, before our range anxiety finally leaves us.