The massive 300-550 kg battery packs that go into electric cars are probably the most important component by far, just like the importance of an internal combustion engine to a traditional car. However, the journey that these lithium-ion batteries make when being produced is a very interesting one: from multiple (sometimes unsafe) mines in far-off countries to being packaged into a powerful, high capacity battery which can drive a car forward at very high speeds.
So how exactly are these lithium-ion batteries for electric cars made? The short answer is that a number of rare metals need to be dug out of the earth from various mines. These are then packaged into small individual battery cells (alongside other materials such as plastic, aluminum, and steel), before themselves being packed into battery modules. The end result is a battery pack which is made up of multiple battery modules, a cooling system/mechanism and a small electrical power management system.
Let’s explore some of this in more detail below!
Battery Structure And Necessary Raw Materials
Before we can go into exactly how electric car batteries are produced, it is worth talking about the battery structure and the materials that go into them. Okay, so pretty much all modern electric cars use lithium-ion batteries, which are rechargeable and contain lots of lithium atoms which can be electrically charged and discharged (known as an ion).
A fully charged battery will have the ions at the negative electrode (the cathode), which will transfer to the positive electrode (the anode) when they have been discharged (i.e. used up). When you plug your EV in to charge back up, the ions move back to the negative electrode, restoring the car’s battery capacity and therefore driving range.
This particular movement of ions occurs inside an individual battery cell, similar to the battery inside your cell phone. However an electric car naturally has much more power than a single cell could provide, so multiple battery cells are grouped together into a battery module (where the cells are arranged in a frame to protect from vibrations and heat build-up).
Finally, multiple modules are organised into the overall battery pack which is installed in electric cars. Every car manufacturer uses different numbers of cells and modules within the pack, but as an example the BMW i3 has a total of 96 battery cells across 8 modules (12 cells per module, and 8 modules per pack):
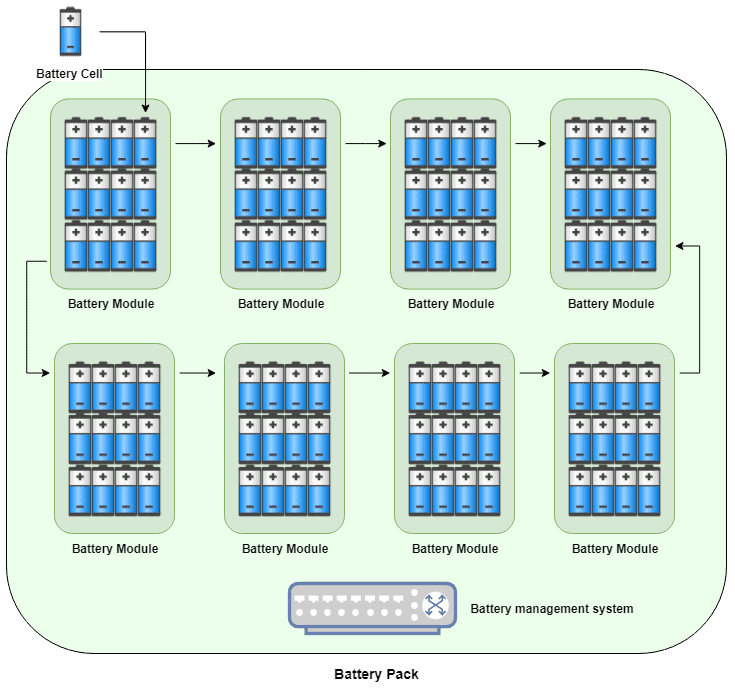
Materials Within A Battery Cell
In general, a battery cell is made up of an anode, cathode, separator and electrolyte which are packaged into an aluminium case.
The positive anode tends to be made up of graphite which is then coated in copper foil giving the distinctive reddish-brown color.
The negative cathode has sometimes used aluminium in the past, but nowadays is a mix of cobalt, nickel and manganese leading to a mixed-metal oxide material.
The electrolyte is a lithium salt in a dissolved solution known as a solvent (which is usually ethylene carbonate). Finally, the separator (which – as its name suggests – physically separates the anode and cathode) is a micro-porous compound which is usually plastic-based such as polypropylene or polyethylene.
Materials Within A Battery Module
As mentioned earlier, the module houses the individual cells and protects them from vibration and heat. The main container typically uses a mix of aluminium or steel, and also plastic.
The individual battery cells within the module need protection from heat and vibration, so a number of resins are used to provide mechanical reinforcement to the cells within the module:
- Epoxy
- Urethane
- Silicone
- Acrylic
- Polyester
Materials Within A Battery Pack

The battery pack’s housing container will use a mix of aluminium or steel, and also plastic (just like the modules). The battery pack also includes a battery management (power) system which is a simple but effective electrical item, meaning it will have a circuit board (made of silicon), wires to/from it (made of copper wire and PVC plastic for the insulation), and resistors/capacitors which use a mix of materials:
- Metals like copper, nickel, lead, tin and aluminium
- Plastics for the housing like PVC, PET and polystyrene.
Phew, we have really drilled down into the raw materials at play here! One of the things you may have realized is that plastic such as PVC/PET and metals such as steel/copper/aluminium are very common and thus these are not a massive issue for car manufacturers to source: their supply is plentiful both locally and internationally.
However some of the rarer metals that make up a battery cell (like lithium and cobalt) are not as easy to source, and the rapid rise of electric cars means that greater pressure is placed on rare earth miners than in the past. The next chapter examine how some of these rare metals are mined, so that the battery cells can be manufactured.
How Rare Metals Are Mined
The process of mining the rare metals varies depending on the mine, however our ‘Electric Cars Aren’t Green?’ sums up how some of the mines operate:
At a mine in Jiangxi, China, workers use ammonium sulfate poured into big holes to dissolve the clay.
- What’s left is hauled out of the ever-expanding hole, before being run through multiple acid baths to dissolve other unwanted compounds.
- The resulting compounds are baked in a kiln, finally revealing the rare metals required in electric car batteries.
- Just 0.2% of the result is the rare metals; the other 99.8% is waste.
- This 99.8% waste earth (and other compounds) – which is now contaminated with toxic material – is dumped back into the originally-created holes.
Many of these rare earth mining processes also unleashes plumes of sulphur dioxide into the atmosphere, and can harm aquatic life in nearby rivers and streams too. Finally, 50-60% of cobalt comes from the Congo, which unfortunately has a poor human rights record with 40,000 children working in cobalt mines for $1-2 per day.
The reason we are mentioning this in a “making of…” article is because car manufacturers are rightly concerned about the chemical composition of their EV batteries, and they are working on tweaking the metal composition to reduce the dependence on some of the worse metals such as cobalt and nickel. Hence the material list that we mentioned towards the start of this article might (or hopefully!) start to change throughout 2020-2030.
Making The Battery Cell
The first thing to point out is that a battery cell which goes into an electric car is not a round, circular battery like we use in our home electrics (and not like the one in our diagram earlier!). A round battery would not be a very efficient use of space (since the car might have hundreds of them in its battery pack) – so instead a flat-cell battery cell is produced.
This is made up of multiple sheets of cathode, anode and separator – which can then be stacked on top of each other like the filling in a Subway! This end result is a cubic shape which is then packaged into an aluminium and plastic frame.
With that said, let’s back up a bit and drill down into the detail. The first thing is to create the sheets of cathode and anode, which arrive in the factory as a big metallic calender roll (just like a big master roll of carpet):
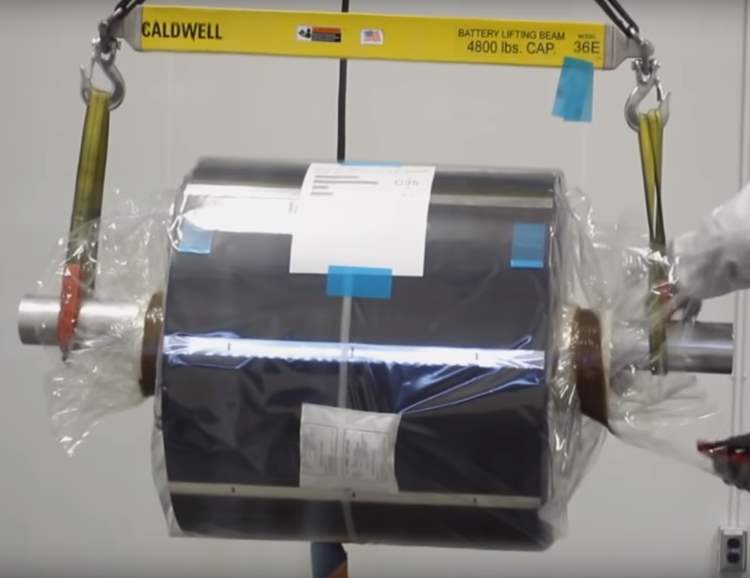
(thanks to the handy video from MotoManTV)
This then goes through a set of processes:
- Mixing: creating the lithium slurry compound via giant mixers.
- Coating: the lithium compound is applied to the metallic roll.
- Drying: the resulting electrode roll is then dried in very high temperatures (and very low humidity conditions), which reduces cracking of the surface material.
- Pressing: a roll pressing machine is then used to repeatedly apply pressure to the roll, which increases its density and thus gives it higher energy/power capacity per volume, along with improve electric conductivity and material adhesiveness.
- Slitting/Cutting: the final worked sheets are cut down to the required length with highly precise cutting tools to avoid frayed edges, and they will be cut small enough to fit into the final battery cell.
At this point we end up with a flat ‘electrode sheet’ (known as a cell layer). These individual cell layers are then stacked on top of each other in the form of anode, separator and then cathode to end with up:

(thanks to the handy video from Lithium Battery Company)
Each layer is connected with a tab, which is what you can see sticking out on the image above. These tabs are stuck or welded together, and the layers are laminated with aluminium foil. Finally, the liquid electrolyte solution is injected in-between the layers which allow the ions to travel between the two electrodes.
At this point, the flat battery cell is finished. We have a fairly durable item which can charge and discharge as required. However they cannot go straight into a battery module and the final product: like a good cheese or wine [or both together: aged cheese and wine go great together… but back to the article!], the cell needs to be aged over time. They are also charged and discharged to activate the cell regularly and ensure its electrical properties are as expected.
Creating A Battery Module
Just like cell layers were stacked on top of each other to create a battery cell, the finalised battery cells are then stacked on top of each other within a metal (aluminium/steel) or plastic frame which is purpose built.
As mentioned earlier, the module protects the individual cells from both heat and vibrations. This is achieved by applying resins and/or thermal interface material between each cell layer (like we have cheese or sauce between the main ingredients of a sandwich!). There might also be more active cooling mechanisms applied, hence the necessary materials (as we discuss later) will be fitted in-between the cells as well.
Every car manufacturer has a different number of battery cells per battery module: some might have just four cells per module, leading to a module which looks like:

(thanks to the handy video from MotoManTV)
Other car manufacturers may have more cells per module, or they might have much larger battery cells leading to much larger battery modules (and then fewer modules in the battery pack):
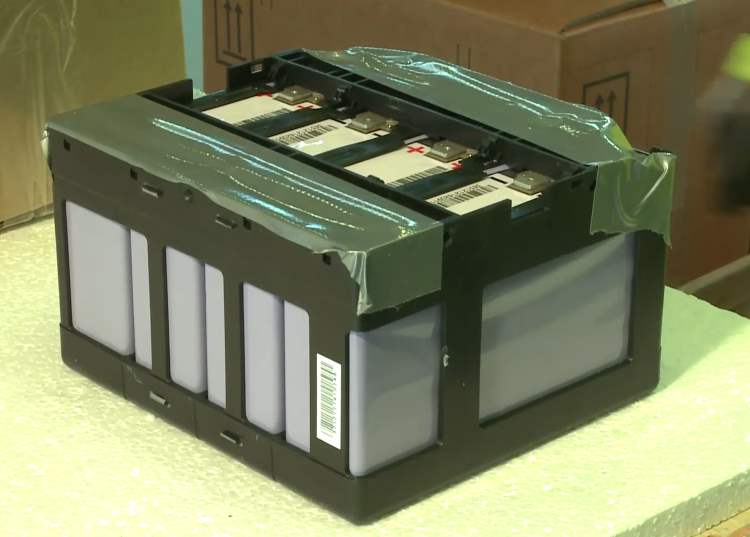
(thanks to this handy video – ignore the packing tape along the top edges though; this is because the battery is being packed for return, not installation!)
The exact design of the battery (and the composition of cells and modules in the pack) is constantly evolving, but the basic idea is that the battery module helps to protect the all-important cells from heat and vibrations/movements.
Producing The Battery Management (Power) System
At this point we have lots of battery modules, packed with all the power capacity that will be needed to move the car forward. However it would not be safe purely to hook this up to the motor controller and hope for the best: we need to ensure that the battery is safe in all conditions, and does not give out too much or too little power in any given situation (otherwise the battery and other car components could get damaged).
The CEO of Daimler (who produce Mercedez-Benz and SMART cars) famously said in 2017 that:
“The intelligence of the battery does not lie in the cell but in the complex battery [management] system,”.
Daimler CEO, 2017
In other words, we need a smart electrical component that can manage the huge amount of power that the EV’s battery is storing away.
The actual BMS module will be a standard electrical component, with a PCB using silicon, and it will have a range of resistors and transistors (made from electrically conductive metal and plastic housing). Naturally every car manufacturer will use their own BMS system, although an example to look at if you want more detailed information is the 12-Cell Lithium BMS Module from Zera (which is aimed more at EV ‘DIY’ conversions, but the same principles apply to mainstream EVs). A general BMS module will look something like:

The battery/power management system is wired up to each battery module, allowing for the state of charge and state of capacity to be measured, along with a host of other safety and management measures. If you are interesting in electrics, then Renesas have a very handy guide on the specifics of a BMS.
Battery Cooling System Construction
Have you ever felt how hot your smart phone gets when it is installing loads of updates, or it is being charged? Well that is with a single battery cell: imagine what happens when you have a hundred or more battery cells, all working to move a car along a road really fast! As you can guess, this generates a lot of heat and the battery cells need to be protected from this.
There are a few ways of doing this. We mentioned earlier on about resins and/or TIM being applied between battery cells. This is one approach, although some car manufacturers apply additional methods when building the battery module (and ultimately the pack):
- Metal cooling plate: this is where a stainless steel heat exchanger unit is applied to the underside of the battery modules (hence the underside of the cells), and the heat can be moved along the plate – away from the cells.
- Thermal fins: if you have seen inside a computer, you will have seen lots of metal fins starting at the CPU (the brains of the computer) and moving away from it, usually with a fan attached to this unit. This is an example of thermal fins, which are thin pieces of metal which help to pick up heat from a heat source (like a battery cell or a CPU) and transfer it across its fins, away from that heat source.
- Water cooling: the Tesla Model S has coils of water pipes throughout the battery pack, and this contains a water-glycol solution which removes heat from the underside of the cells and provides cooling to them instead. This is a similar approach to high-end computer systems which are water-coolant cooled via a network of pipes and a pump.
Putting It All Together: The Battery Pack
Finally, we have the battery pack: where the battery cells (contained in the modules) are all housed together, cooled via the cooling system and managed by the power management system (and the motor controller, a separate component to the battery). The overall frame will be made from a mix of metal (usually steel or aluminium) and also plastic. The various components will be welded and/or bolted into place. The end result might look like the following Tesla batteries:

Or like the following mock-up of a Nissan Leaf (with the plastic housing partially cut away):

Not green or inexpensive.
Thank You. I had no idea so, for me, it was very educational. A Well Done Basic Introduction. The only question I was left with was battery life. In case any of your other readers have the same question I looked it up. Basically all examples go well over 100K. At the end of their use they run down incrementally. One example: the new Lexus UX300e, which is the first vehicle to feature a 10-year, 1 million kilometer warranty on the battery pack. If that’s not confidence in the technology, we don’t know what is. Thanks Again.
Of course the idea that the rest of car will last 625,000 miles does affect the return on investment in terms of fuel savings.
When projecting expense you have to account for the increased value of a vehicle you could reasonably expect to be driving for the next 17 years with reduced fuel and maintenance costs. Ie: the new Lexus UX300e, which is the first vehicle to feature a 10-year, 1 million kilometer warranty on the battery pack. If that’s not confidence in the technology, we don’t know what is.
Great information, simple and concise. Thank you. I have bookmarked this for future reference. I think I’ll send a link to all my ‘electric car’ fanatics so they can understand just this one problem with their beloved toys.
A very deceptive and incomplete answer to an important question. Let me go buy another diesel — works for me.
So curious, and this maybe a silly question. A normal car battery will lose 30- 50% of its capacity when the temperature falls below freezing. Does cooler weather affect this type of battery?
Good question, definitely not a silly one. The EV battery itself won’t lose range purely due to the cold weather (i.e. not from a physical perspective), BUT the extra demands of running the car in cold weather – e.g. extra heating – WILL deplete the battery. So it’s similar to a gasoline car in that regard.
The internal combustion engine provides the heat provided in a conventional vehicle via the coolant. Essentially you have a small radiator behind your dash. When you turn the thermostat to heat, it opens a valve that allows the hot coolant to flow through that circuit. In essence, you are utilizing the heat already being generated from the combustion process. EVs utilize electric heaters, so they consume more electricity from the battery cells, taking away from your range. So you can not compare the heating provided by an electric vehicle to that of an internal combustion vehicle.
Interesting that the comments so far deal with battery life primarily.
In the meantime, China is releasing more toxins into the air /ground/water with their efforts to get the raw materials and the Congo is using CHILDREN that are paid a dollar or two a day. So we are talking about human rights violations and toxins to get a vehicle that has a questionable effect on the environment but may save the electric car user a few bucks a gallon on the artificially set prices at the pump.
Just so we are clear.
My first Prius was a 2012 Prius-C . The first cold spell we had, I took the car back to the dealer. The miles per gallon dropped significantly. They said it was the cold weather. I did not know, the cold does matter.
Environmentally speaking, gasoline and electric powered cars need materials gained from mining/earth endangering processes. Do you know which is worse overall? Can you direct me to non biased studies?
Hi Carole, yes you’re right to flag this up. I talk about this in another blog post (https://www.greencarfuture.com/electric/are-evs-harmful-for-environment) although the gist is that EVs are generally better than gasoline cars, although this certainly isn’t true for the first ten thousand miles or so. This is improving though, with less rare earth metals being used in EV battery packs.
Thanks
Really? EVs are way worse than an ice car. I read in another article that children were down in the mines. All the green ppl in the USA don’t care about that, huh?
And if I read the article correctly, the battery packs are made in China? And the minerals needed to make the battery are mined in third world countries? And how about the disposal of the battery packs and cars for that matter? I recently read of a “boneyard” of electric cars I believe in Norway, that cannot be disposed of. And another question, is oil and gas required to make these products as well as everything else that is produced?
Thank you for any insight you can provide.
It’s hard to say specifically because many EV manufacturers source their batteries from different companies, and even Tesla source their batteries in multiple ways. But in short, many battery packs do require minerals mined from third world countries, yes: this is something many EV companies are trying to improve on, but right now it’s the reality.
Also yes, a fair amount of oil/gas would be used during the manufacturing pipeline. Overall, though, EVs are still a net benefit on the environment compared to gasoline cars: https://www.greencarfuture.com/electric/are-evs-harmful-for-environment
Explain this part of the Great Green Batteries!
Exide cleanup: Newsom budget proposes $454M for Vernon battery plant site
ByABC7.com staff KABC logo
Monday, May 17, 2021
Newsom budget provides $454M for Exide plant cleanup
EMBED More Videos
Gov. Gavin Newsom has proposed $454 million for cleaning up lead contamination at the former Exide plant in Vernon.
VERNON, Calif. (KABC) — A decades-long battle to clean up contamination in Vernon and surrounding communities is getting a new boost from Gov. Gavin Newsom’s proposed budget.
Neighbors and activists have been fighting for years to clean up contamination at the Exide Technologies battery recycling plant in Vernon.
Newsom has included $454 million in his state budget proposal to clean up the polluted homes and neighborhoods.
“No one should have to slowly see their family members dying in excruciating pain,” said Boyle Heights resident and activist Terry Gonzalez-Cano. “Or their children not be able to have children, or not even be able to live long enough to see them graduate. We need this money.”
Gonzalez-Cano lost both of her parents, several neighbors and friends to illnesses caused by toxic levels of lead contamination linked to the plant.
More than 10,000 homes in the communities of Bell, Boyle Heights, East Los Angeles and Vernon were polluted by the former Exide battery recycling facility.
“This is our very own Flint, Michigan,” said Los Angeles City Councilman Kevin de Leon, referring to the water contamination scandal in that city. “You don’t have to go to the Midwest to find lead poisoning and contamination. It’s in our own very backyard.”
Local leaders say Exide Technologies’ corporate greed allowed the pollution to materialize for years. And they blame the state’s Department of Toxic Substances and Control for failing to take action.
Last fall a judge approved Exide’s bankruptcy settlement, allowing the company to abandon the plant and leaving taxpayers responsible for footing the cleanup.
Neighbors say they will continue to raise their voices until the nearly half-billion dollar cleanup is approved.
Not true. EV just push the pollution it would have created to the Power Generation Plants.
But we are dependent on China for these resources & thanks to Hunter Biden who helped china buy one of the richest cobalt mines.
“Hunter Biden, the president’s son, was a founding board member helped facilitate a Chinese company’s purchase from an American company of one of the world’s richest cobalt mines, located in the Democratic Republic of Congo” So its ok for them to work slaves, & children & pollute their land so we can feel good about being green. Meanwhile China is controlling the market and building coal plants at an accelerated rate.
In the long run we see rapid transit becoming more needed,
worldwide,than electric and gas powered private vehicles,
since the cost of battery replacement,is beyond the reach,
of 2nd and 3rd time buyers.
In My opinion, gasoline/electric hybrids are the best solution at this point.
what about the supposed new “Glass Battery” ? is it real or just another financial scam?
Right now, no-one seems to know for sure. Tesla/Musk haven’t spoke about this tones, and certainly haven’t announced any major move to “glass batteries”. But who knows – maybe Battery Day 2022 will unveil this? My hunch is that it’s a promising idea, but it’s not just ready for mainstream EVs.
If, say even 50% of all cars were electric what would be the additional demands on electric power generation?
From mining and processing of the iron ore for the 1.5 inch thick high grade steel towers to the mining and transport and MELTING of thousands of tons of sand into the glass fibers for the gigantic blades and the tons of resins and epoxies to form and cure the blades to the miles of fine copper windings for the turbines generators and connection to the grid to the mining and crushing and processing thousands of tons of limestone and portland cement into 50 ton concrete bases and fuel for transport and erection what is the total carbon footprint to create and put into service one 3rd generation wind turbine? How long does it have to operate IN REAL WORLD TIME AND NUMBERS TO BECOME CARBON NUETRAL AND HOW MUCH LONGER AFTER THAT UNTIL REQUIRED DECOMMISSION AND DISASSEMBLY? SEE NPR ARTICLE ON “‘WASTE PROBLEM WITH WIND’.
A fair comment, thanks for your insight. It does seem like one of those things that might “get worse before it gets better” (although naturally the last part is arguable). Here in the UK, electric prices are sky-high – much higher than in America. And the Government has (rightly, IMO) scrapped the EV subsidy. So it means that buying an EV costs quite a lot (£40-60k, sometimes) and then we pay sky-high electric prices to charge it.
That doesn’t begin to cover the points you make about the environmental impact of extra green energy power, either.
So I do agree with some of your points – EVs right now don’t seem completely ready for the mainstream, even though I’m naturally more positive about them than many are.
My question is is it more beneficial in the long run to buy an Ev given how much environmental damage and mining techniques that is going into make the batteries etc
It’s definitely a fair concern Bill. In general they are better than gasoline cars when driven for tens of thousands of miles (I cover this a bit more in another article), but for occasional drivers, a cheap second hand gas car will probably be better for the environment than a brand new EV.
I have worked for the united nations environment programme and am a full supporter of renewable energy and green cars. But electric cars can be considered green only if the electricity they use comes from renewable sources. What percentage of electricity used by electric cars comes from renewable sources today ? If the electricity used by electric cars comes from burning coal and fossil fuels, then electric cars cannot be considered green. Let us first concentrate on using the sun, wind, geothermal, hydro and the sea to produce our electricity before concluding that electric cars are green. And what about splitting sea water into hydrogen and oxygen using solar energy (sunlight) and burning the hydrogen to produce our electricity ? Something everyone should be hydrogen on !!!
Hello Dr Reuben Shanthikumar, thanks for your comment. I totally agree that ‘eco-friendly’ electric cars aren’t really eco-friendly if their fuel source comes from fossil fuels. It’s a little tricky because, in reality, it’s a mix: some homes (and charging stations) are solar powered, but others will ultimately source their electric from fossil fuels. So it’s possible that an EV has a mix of eco-friendly fueling sources.
I do however agree with your general point though: the world still has a long way to go to become properly eco-friendly and self-sufficient.
To Piggy-back off of Dr Reuben Shanthikumar question, if you are able to produce your own electricity to supply your EV, greater saving will be realized. I have a solar system on my house suppling the energy needed to “refuel” my EV which greatly amps up the savings of the operation over am other ICE vehicle.
BTW, great article.
Thank you for putting this together, very informative. I am not quite understanding why our government is pushing EV’s when the battery technology is not quite there and our power grids cant handle extra load (solar and wind have their own negative effects) Seems like we are substituting one problem for another. I wish more environmentalist would consider the benefits of fossil fuel energy vs the negatives. Millions and millions of people would not survive without it. I very much understand the need for clean energy but we cant look at it in a vacuum.
No problem, and I agree with everything you’ve said. Naturally I’m an EV fan, but they are not a magical panacea for enviromental issues. Even Elon Musk has recently said that the world would crumble without oil and gas.
Follow the money
What percentage of the battery is used to run the vehicle and at the same time the percentage to maintain the use of the battery itself?
The exact percentage for running the EV is pretty high – over 90% in most cases. And the opposite is true for your second question – only a tiny percentage of power is used to maintain the battery itself (less than 1% per day).
I have been concerned at the lack of interest by the government, persuing the green agenda, in the enviromental harm the mining and use of rare metals causes. Politicians of all parties seem to jump on the green bandwagon without due consideration of factors that argue for continued use of exsisting fuels until the new technologies are actually going to do less harm.
As a pensioner I am unlikely to be able to afford an ev or hybrid car!
But we are dependent on China for these resources & thanks to Hunter Biden who helped china buy one of the richest cobalt mines.
“Hunter Biden, the president’s son, was a founding board member helped facilitate a Chinese company’s purchase from an American company of one of the world’s richest cobalt mines, located in the Democratic Republic of Congo” So its ok for them to work slaves, & children & pollute their land so we can feel good about being green. Meanwhile China is controlling the market and building coal plants at an accelerated rate.
rfgdrgvdscdsa